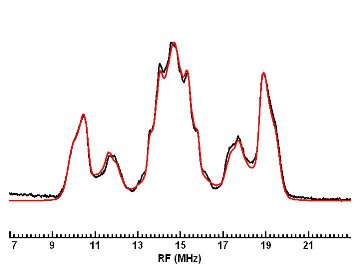
ENDOR (Electron-Nuclear DOuble Resonance) spectroscopy is an EMR technique that often is described as EPR-detected NMR. It is very useful for learning about the structure of paramagnetic molecules, as well as information about the distances and orientations of atoms surrounding paramagnetic centers (e.g. proteins and enzymes containing paramagnetic ions, and defects in solids). ENDOR makes use of the electron-nuclear hyperfine couplings (I . A . S) between the unpaired electrons and neighboring nuclei with I >= 0, and measures them with much higher precision than may be possible by EPR spectroscopy alone. Because it examines nuclei in the vicinity of unpaired electrons, ENDOR can provide information that conventional NMR misses, due to the short relaxation times of these nuclei. The technique uses two frequencies: a fixed microwave frequency to partially saturate electronic Zeeman transitions and monitor the intensity of the EPR signal, and a strong radiofrequency which is varied in order to excite nuclear (NMR) Zeeman transitions. At a magnetic field (Bo) of 0.34 T, the microwave frequency for a g=2 paramagnetic species would be about 9.5 GHz, while radiofrequencies suitable for driving proton NMR transitions would center around 14 MHz. By driving NMR transitions with the strong rf field, a particular nuclear transition rate is enhanced. This is communicated to the electrons by means of the hyperfine coupling between that nucleus and the electron, increasing its induced relaxation rate, which changes the EPR signal intensity of these partially saturated electronic transitions. In this way, ENDOR reports on the NMR of nuclei in the vicinity of paramagnetic electrons.
ENDOR spectroscopy usually is performed on paramagnetic species in liquids and solids. In liquids, ENDOR spectra typically give information on the isotropic hyperfine couplings, while in solids (single crystals, powders, frozen solutions) both isotropic and anisotropic hyperfine interactions are observed. The analysis of ENDOR spectra requires a careful computer simulation in order to determine the precise values of the hyperfine interactions. This information, which may take the form of a matrix for each nucleus, can, in turn, be analyzed to determine the distances and orientations of I != 0 nuclei in the vicinity of the paramagnetic electrons. In solid systems with electronic Zeeman anisotropy, additional information is available from the Bo dependence of the ENDOR spectra, a phenomenon known as "orientation selection." The ability to perform ENDOR at several different magnetic fields is very useful in studying systems of this type (which include most proteins and enzymes that contain transition metal ions, for example), as well as for characterizing the effects of motion on ENDOR spectra. Because the rates of electronic and nuclear transitions must be made comparable in order to observe a strong ENDOR effect, the technique also requires precise temperature control, often at very low (liquid helium) temperatures.
While most ENDOR experiments in the past used a CW microwave source, more and more pulse ENDOR experiments are performed today. Because of the low RF duty cycle, pulsed ENDOR does not have the problems of RF heating seen in CW ENDOR. Also, by varing pulse widths and times, one can select different spins, depending upon their hyperfine coupling and relaxation times. Generally, there are two types of pulse ENDOR experiments, Davies and Mims, which are named after their originators. Both methods require the observation of a spin echo, but have different pulse sequences. Mims ENDOR is more sensitive to small couplings, but is less quantitative due to blind spots. Davies ENDOR is better for larger couplings. Davies ENDOR can have effeciencies close to one unlike the case for CW ENDOR where effeciencies are small and difficult to quantitative[1].
The EPR Lab has experience performing ENDOR on paramagnetic molecules [2,3], as well as on frozen solutions of proteins [4], to mention two examples from past studies. Initially we used primarily CW ENDOR, but now the EPR Lab is primarily using pulsed ENDOR at X-band [5,6]. We now have the ability to perform ENDOR at 35 GHz (Q-band). The Lab has developed computer simulation techniques for the analysis of ENDOR spectra from disordered solids, including spectra exhibiting "orientation selection," as well as for S >= 1/2 systems [6].
References
- C. Gemperle and A. Schweiger, "Pulsed electron-nuclear double resonance methodology", Chem. Rev. 91, 1481 (1991).
- "ENDOR of Perylene Radicals Adsorbed on Alumina and Silica-Alumina Powders. I. The Ring Protons", R. B. Clarkson, R. L. Belford, K. Rothenberger, and H. Crookham, J. Catalysis, 106, 500 (1987).
- "ENDOR of Perylene Radicals Adsorbed on Alumina and Silica-Alumina Powders, II. Matrix ENDOR", K. Rothenberger, H. Crookham, R. B. Clarkson, R. L. Belford, J. Catalysis, 115, 430 (1989).
- "Nitrogen and Proton ENDOR of Cytochrome d, Hemin, and Metmyoglobin in Frozen Solutions", F. Jiang, T. M. Zuberi, J. B. Cornelius, R. B. Clarkson, R. B. Gennis, and R. L. Belford, J. Am. Chem. Soc., 115, 10293-10299 (1993).
- Yap, L.-L., Samoilova, R.I., Gennis, R.B., Dikanov, S. A. (2006) Characterization of the exchangeable protons in the immediate vicinity of the semiquinone radical at the QH site of the cytochrome bo3 from Escherichia coli. J. Biol. Chem. 281, 16879-16887.
- B. Barquera, L. Ramirez-Silva, J. E. Morgan, and M. J. Nilges, ‘A new flavin radical signal in the Na+-pumping NADH: Quinone oxidoreductase from Vibrio cholerae: An EPR/ENDOR investigation of the role of the covalently bound flavins in subunits B and C,” J. Biol. Chem., 281, 36482-36491(2006).
The orginal text was written by Robert B. Clarkson; update by MJN 5/2008.